By the way, recently I have had the occasion of testing some of the K-Fe oxide material at high temperature under flowing H2 inside a pencil-sized furnace open to the atmosphere. The flow was such that the H2 combusted near the opening, also catalytically at the iron oxide material put there.
Under these conditions I haven't been able to observe heat that was not clearly associated with H2-O2 recombination, unfortunately, and the material located more inside the tube did not seem to show increased temperatures from the hydrogen flow (not to any visible extent at least). The tests however were as usual relatively crude—I was just looking for large-scale changes—and the H2 gas from electrolysis was not dried. Moreover, it's unclear whether cluster formation takes places at pressures close to atmospheric under (still) relatively low hydrogen density conditions.
Below is an example of the observed changes without (left) and with (right) hydrogen flow:
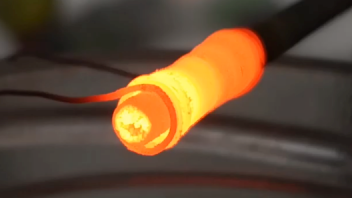
At higher temperatures the emission of potassium from the material was such that apparently it started producing a faint potassium flame (visible on the left photo below), which was markedly different from that of H2 combustion more often visible at lower temperature and/or by increasing H2 flow (right):
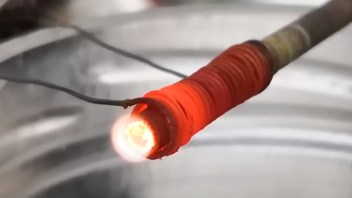
The heating wire was made of Kanthal A1 and the tube of SS304 alloy. They didn't short-circuit due to the thick oxide layer intentionally formed on their surface.
EDIT: earlier I also tried to plug the opening with the same catalyst material, but it turned out to almost completely block hydrogen flow, and no additional heating was seen at all from H2 admission.
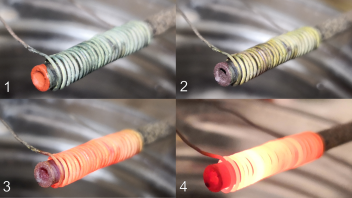
The Kanthal wire looked green at low temperature due to the formation on its surface of KFeO2 due to KOH solution application and heat.
EDIT: here is also a short animation of the previously mentioned apparent potassium flame following hydrogen flow and higher temperatures. I think the volatilized potassium reacted with oxygen more readily than hydrogen would and prevented a "proper" hydrogen flame to form. I could be wrong, though.
